We are witnessing the advent of the New Space Race – and this time, when we go back to space, we’re going to stay.
That makes the question of how to sustain human life in the inhospitable environment of space more pertinent than ever. The challenges are many – we need to breathe, eat, drink, and maintain a healthy body and mind, all while millions of miles from Earth. The solution is a sophisticated Environmental Control and Life Support System (ECLSS).
At the core of these space-faring solutions are two primary paradigms – the Physicochemical Life Support Systems (PCLSS) and Bioregenerative Life Support Systems (BLSS), each with a unique set of methodologies, strengths, and weaknesses. Further branching from BLSS is a promising subtype: Closed Ecological Life Support Systems (CELSS), a bold endeavor to create self-sustaining ecosystems within spacecraft – and the preferred approach of The Spring Institute for Forests on the Moon.
Types of Life Support Systems
In PCLSS, physical and chemical processes drive the recycling of air, water, and waste. The process is efficient, usually reliable, and rapid, although not indefinitely sustainable. On the other hand, BLSS uses living organisms – plants, algae, and microbes, for example – to regenerate life-sustaining resources. They operate more slowly, yet hold the promise of long-term sustainability, particularly due to the adaptability of living systems to changing environments.
Taking it a step further, CELSS aims to mimic Earth’s own biosphere, integrating a (bio)diverse array of living and non-living elements. Just as ecology encompasses the relationship between plants, animals, and microbes with their environment (including as soil, air, and water), a CELSS uses natural processes to create a harmonious, self-regulating environment. Imagine an astronaut tending to an aquaculture rice paddy on Mars, beneficial and tasty carp (or shrimp!) swimming amongst the reeds, and a robust wetland system upstream processing waste. Sounds fantastical, doesn’t it?
In this article, we will delve into the nuances of these life support paradigms, explore how each one addresses the key components of environmental control and life support, and discuss their potential application in future space missions. Whether you’re a seasoned space professional or a curious enthusiast, join us as we embark on this journey to understand the mechanisms that may one day sustain human life on distant worlds.
Components of Environmental Control and Life Support Systems
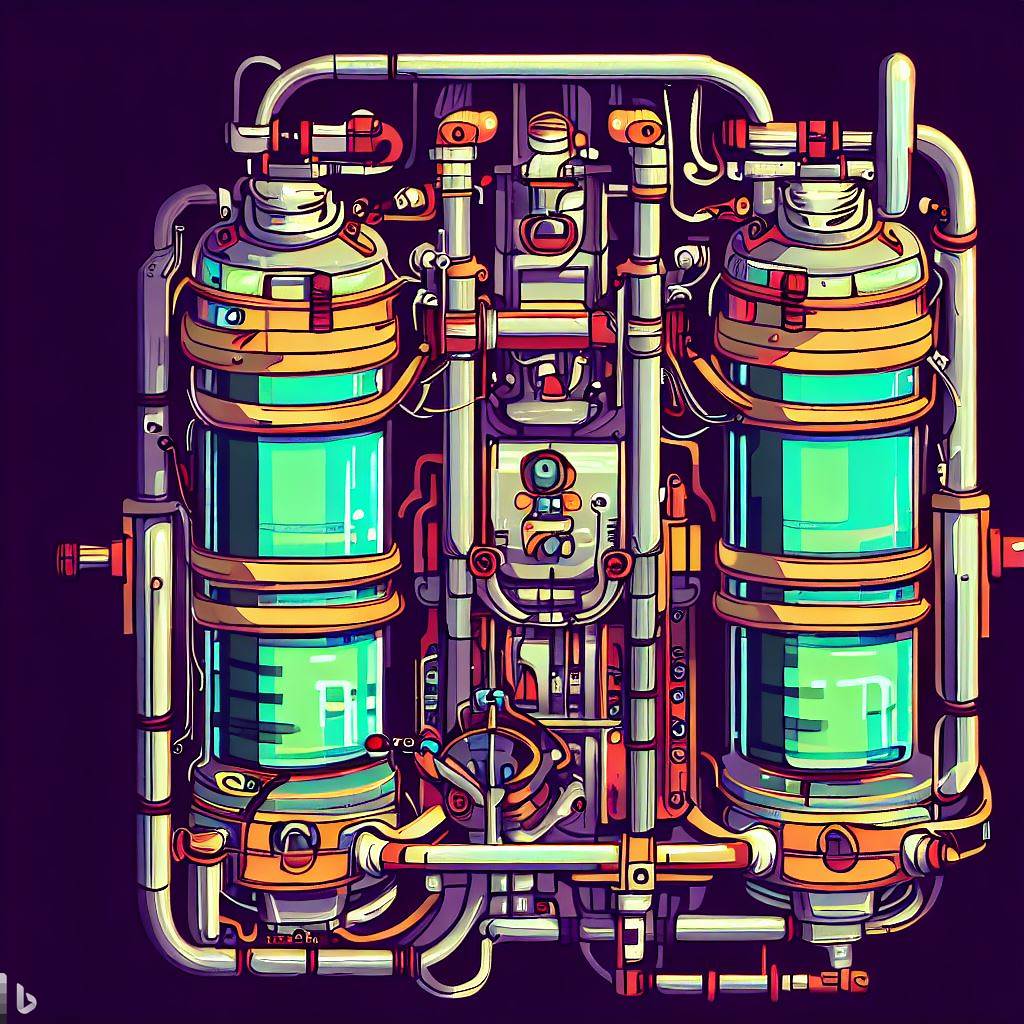
Environmental Control and Life Support Systems (ECLSS) are designed to maintain a habitable environment for astronauts within a spacecraft or space station. These systems provide and maintain all necessary elements for human survival and health during space travel or habitation, often for extended periods. The following are the major components of an ECLSS:
- Atmosphere Control and Supply: This system is responsible for maintaining the composition of the cabin air. It controls oxygen partial pressure and total pressure, trace gases, and particulates. This system also includes fire detection and suppression.
- Oxygen Generation: This system produces oxygen for the crew to breathe. On the International Space Station, the oxygen generation system is a machine that uses the process of electrolysis to split water into hydrogen and oxygen. The oxygen is circulated into the cabin air system, while the hydrogen is vented overboard or used in other systems.
- Carbon Dioxide Removal: You need to remove carbon dioxide exhaled by the crew and prevent its accumulation, which would otherwise be toxic. CO2 removal typically uses adsorbent materials, often a mineral called “zeolite” to bind and store carbon dioxide.
- Water Recovery: This complex system collects, recycles, and purifies water from various sources, including urine, humidity from the air, condensation, and waste water. The water is processed to potable standards for drinking and food preparation, and also to provide water for oxygen generation.
- Waste Management: You need a system is designed to collect and process human waste. The solid waste is often stored for later disposal, while liquid waste is typically filtered back into potable water.
- Temperature and Humidity Control: You need to maintain comfortable and safe temperatures and humidity levels within the spacecraft. It typically includes both active and passive elements, such as heaters, coolers, insulation, and humidity removal equipment.
- Food and Nutrition Supply: While not always considered part of the ECLSS, a plan for bringing or producing ample food is essential for long-duration spaceflight. A balanced diet is necessary to support the health and well-being of the crew.
- Radiation Protection: Depending on duration of the mission, this could be an important part of an ECLSS. The solution would help shield astronauts from harmful solar and cosmic radiation, which can quickly cause a number of health issues, such as cancer.
These components work in conjunction to create and maintain a safe and habitable environment for astronauts in space. Note that the specific design and functionality of an ECLSS can vary significantly depending on the mission requirements, vehicle design, and technological advancements.
So, how are each of these needs met by the different types of ECLSS?
Components of a PCLSS
Let’s use the International Space Station as a model PCLSS:
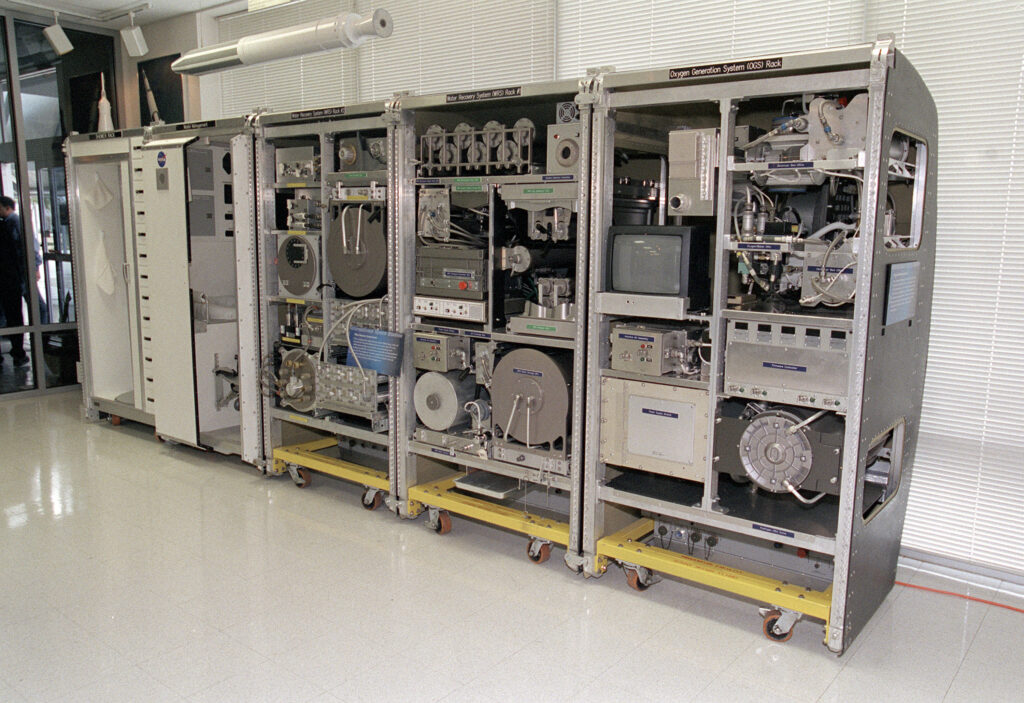
- Atmosphere Control and Supply: The atmosphere is controlled using a combination of gas storage tanks and systems that remove excess gases, like carbon dioxide, through adsorption or scrubbing. The air composition is carefully monitored to ensure it remains stable and within healthy parameters.
- Oxygen Generation: Electrolysis of water is used to generate oxygen, which is then released into the cabin.
- Carbon Dioxide Removal: Physicochemical methods like adsorption are used to remove carbon dioxide from the cabin air.
- Water Recovery System: Wastewater is purified using physical filtration and chemical treatments. Urine is processed using a distillation process to recover water.
- Waste Management: Solid waste is collected, stored, and usually disposed of during a resupply mission. Liquid waste is processed through the WRS.
- Temperature and Humidity Control: Physical cooling and heating units, insulation, and dehumidifiers are used to control temperature and humidity.
- Food and Nutrition Supply: Astronauts are supplied with pre-packaged meals that have a long shelf life.
- Radiation Protection: The spacecraft hull and special shielding materials are used to protect against radiation.
This is the dominant (indeed the only) approach to life support systems currently in use today. These systems are generally faster and more predictable than their bioregenerative counterparts. They require less volume and are generally easier to control.
However, PCLSS have limitations in terms of long-duration missions because they rely on consumables (like filters and chemicals) that would eventually run out and need to be resupplied. Many complex parts cannot currently be manufactured in-situ, and it’s not feasible to bring along many redundant replacements for every possible failure mode.
Components of a BLSS
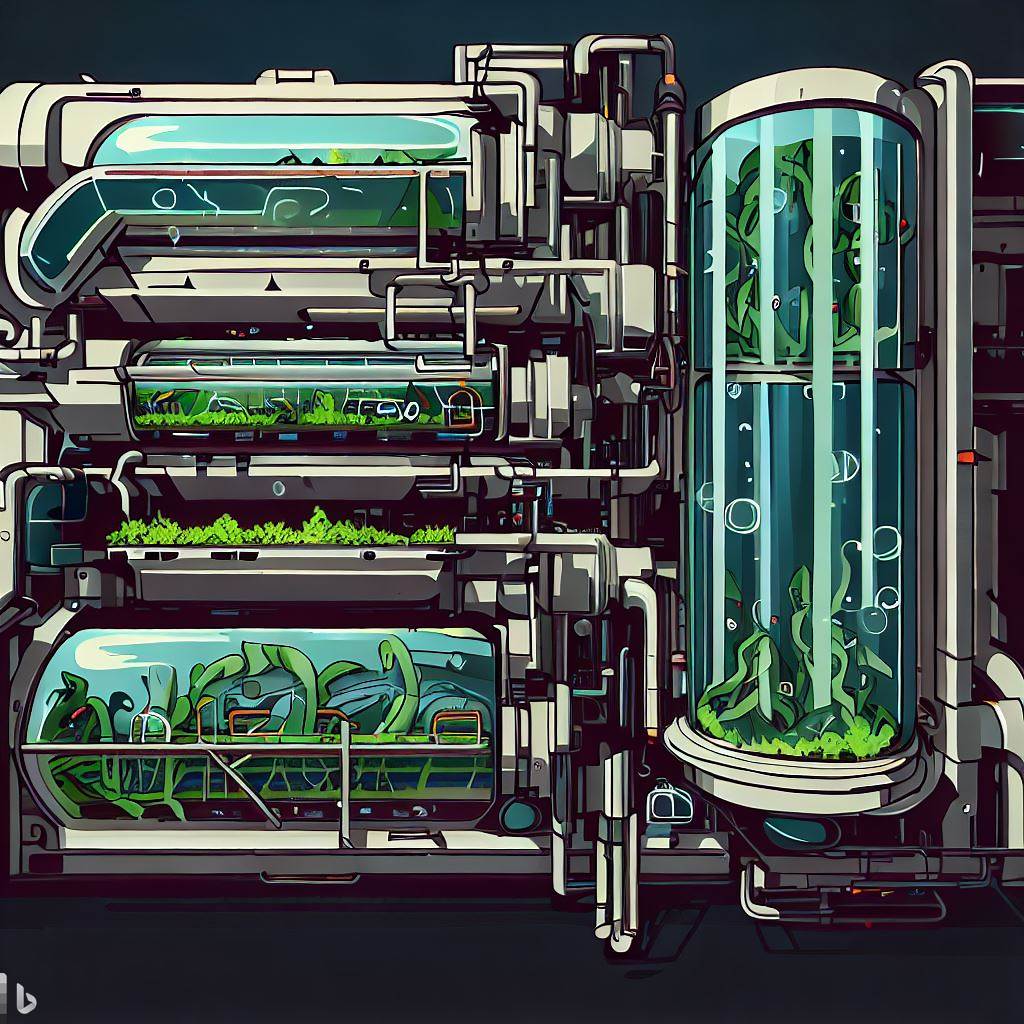
Bioregenerative life support systems, on the other hand, utilize biological processes to regenerate life-sustaining resources. These systems are based on the strengths of individual plants, algae, or microbes, which together make a robust and resilient living approach to human life support in space.
- Atmosphere Control and Supply: Controlling the rate of photosynthesis is a solution for controlling atmosphere composition and pressure, but plants are only able to remove trace amounts of contaminant gases at low densities.
- Oxygen Generation: Oxygen is produced by photosynthesis of plants in a growth chamber, or more likely, with a very efficient algae photobioreactor.
- Carbon Dioxide Removal: Carbon dioxide is absorbed by plants or algae during photosynthesis, and can be sequestered in food or biomass if necessary.
- Water Recovery: Liquid waste such as urine can be applied directly or dilutely to as fertilizer to some plants, or particularly algae bioreactors. It can also be filtered with mechanical and biological filters.
- Waste Management: Solid waste can be composted or broken down by aerobic or anaerobic bacteria, usually in a kind of bioreactor called a “digestor”, and then used as a resource for plant growth.
- Temperature and Humidity Control: There aren’t many good biological solutions to maintaining temperature and humidity. Plants constantly “transpire” water, exhaling humidity in the same way they exhale oxygen.
- Food and Nutrition Supply: Food can be grown directly within the spacecraft, providing a renewable food source. It would likely take place in a controlled agriculture environment, using hydroponics or aeroponics for maximum efficiency.
- Radiation Protection: Biological materials like fungi have been proposed for use as radiation shielding, however, it’s simpler and easier to use a small amount of water as a very effective radiation shield.
BLSS have the potential to be self-sustaining over the long term, reducing the need for resupply missions. They can generate food, recycle water, produce oxygen, and remove carbon dioxide. They can also contribute to psychological well-being by providing adding a splash of green to the astronaut’s environment.
However, BLSS can be slower to respond to changes in demand, more difficult to control, and require more space and light energy. They can also be impacted by diseases, and a lack of continual maintenance could cause the whole system to collapse without proper safeguards.
Components of a CELSS
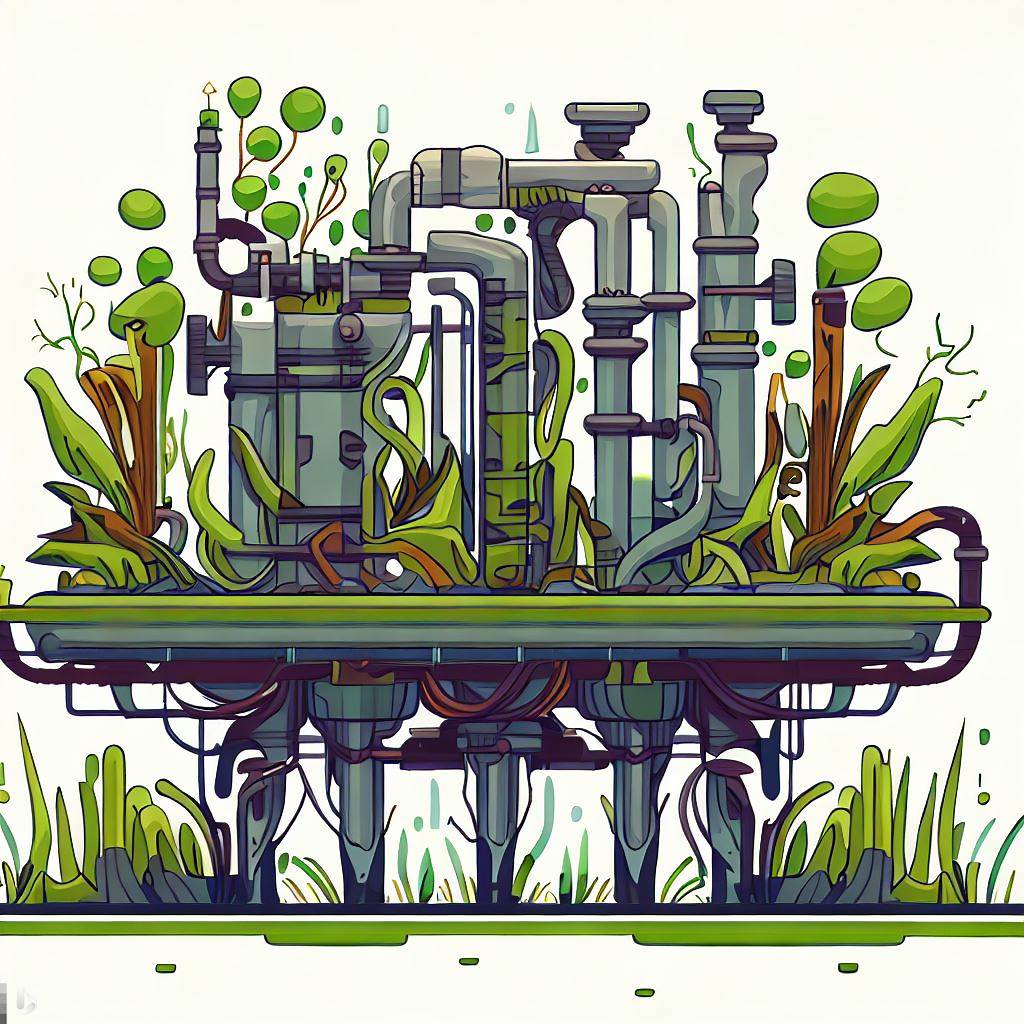
A Closed Ecological Life Support System is a type of BLSS that attempts to create a completely self-sustaining, closed-loop system. It aims to replicate Earth’s biosphere in a miniaturized form to provide the same ecosystem services that our planet provides us: recycling air, water, and waste; as well as producing food, fiber and pharmaceuticals.
While most ecosystem archetypes are capable of performing all of the ecosystem services we are interested in, we’ll continue using the example of a wetland marsh.
- Atmosphere Control and Supply: Again, controlling the rate of photosynthesis is a solution for controlling atmosphere composition and pressure. Unlike the vertical farms of BLSS, however, a marsh produces biomass very rapidly, which would increase the efficiency of plants purifying the air. Even better, the relatively large quantities of soil can act as a soil bed reactor – a microbial air filter that dramatically increases the range and volume of gases processed through natural means.
- Oxygen Generation: Oxygen is easily produced by photosynthesis of plants and algae throughout the marsh. It should be noted that there are a number of biological processes, such as decomposition or animal metabolism, which consume oxygen and will need to be monitored and controlled.
- Carbon Dioxide Removal: Carbon dioxide is absorbed by plants or algae during photosynthesis, and can be sequestered in food or biomass if necessary. Wetlands are particularly adept at sequestering carbon in the form of dead biomass in anoxic zones underwater, preventing decomposition and locking up carbon until such time as it needs to be reintroduced to the system.
- Water Recovery: Liquid waste such as urine can be applied directly or dilutely to as fertilizer to some plants, or particularly algae bioreactors. It can also be filtered with mechanical and biological filters.
- Waste Management: There is a long history of the use of wetlands as natural recyclers of human solid and liquid waste. It’s even been investigated for use in space life support already.
- Temperature and Humidity Control: There aren’t many good biological solutions to maintaining temperature and humidity. Plants constantly “transpire” water, exhaling humidity in the same way they exhale oxygen.
- Food and Nutrition Supply: This is a strength of CELSS. It’s not as maximally efficient at producing edible biomass, like an intensive hydroponic system might be. However, the interconnected ecosystems allow symbioses (like aquaculture) which enhance efficiency and variety and also provide natural recycling of problematic inedible biomass (like plant stems or roots).
- Radiation Protection: Biological materials like fungi have been proposed for use as radiation shielding, however, it’s simpler and easier to use a small amount of water as a very effective radiation shield.
Towards a Sustainable Future with CELSS
As our exploration of space expands, and as we contemplate longer missions and more distant goals, the need for sustainable, self-contained life support systems grows exponentially. Though both PCLSS and BLSS provide valuable strategies for maintaining human life in space, we posit that CELSS hold the most promise for long-term sustainability.
Why does The Spring Institute champion CELSS so fervently? Because CELSS are the only theoretically self-sustaining life support system, based on Earth’s own proven model of a self-regulating, interconnected ecosystem. By replicating the Earth’s natural processes, we can create a harmonious environment that not only supports human life but also promotes psychological well-being.
CELSS is not just about surviving in space. It’s about thriving. About planting and harvesting food, observing the life cycles of bugs and and animals, watching the interaction of a stream and its banks, understanding the constant cycling and recycling that enables the persistence of life. It’s about bringing the best parts of Earth with us wherever we go.
The promise of CELSS is not merely in aiding space exploration, but in enhancing our understanding of our home planet’s delicate ecosystems. By studying and implementing CELSS, we gain insights that can guide our stewardship of Earth, leading to advancements in sustainability and conservation here at home.
In the short term, adopting a CELSS approach will undoubtedly present its unique challenges. Balancing a closed ecosystem is delicate work, requiring intricate knowledge and precise control. It’s full of unanswered questions, and might ultimately not even be possible at a human-feasible scale. But we have to try.
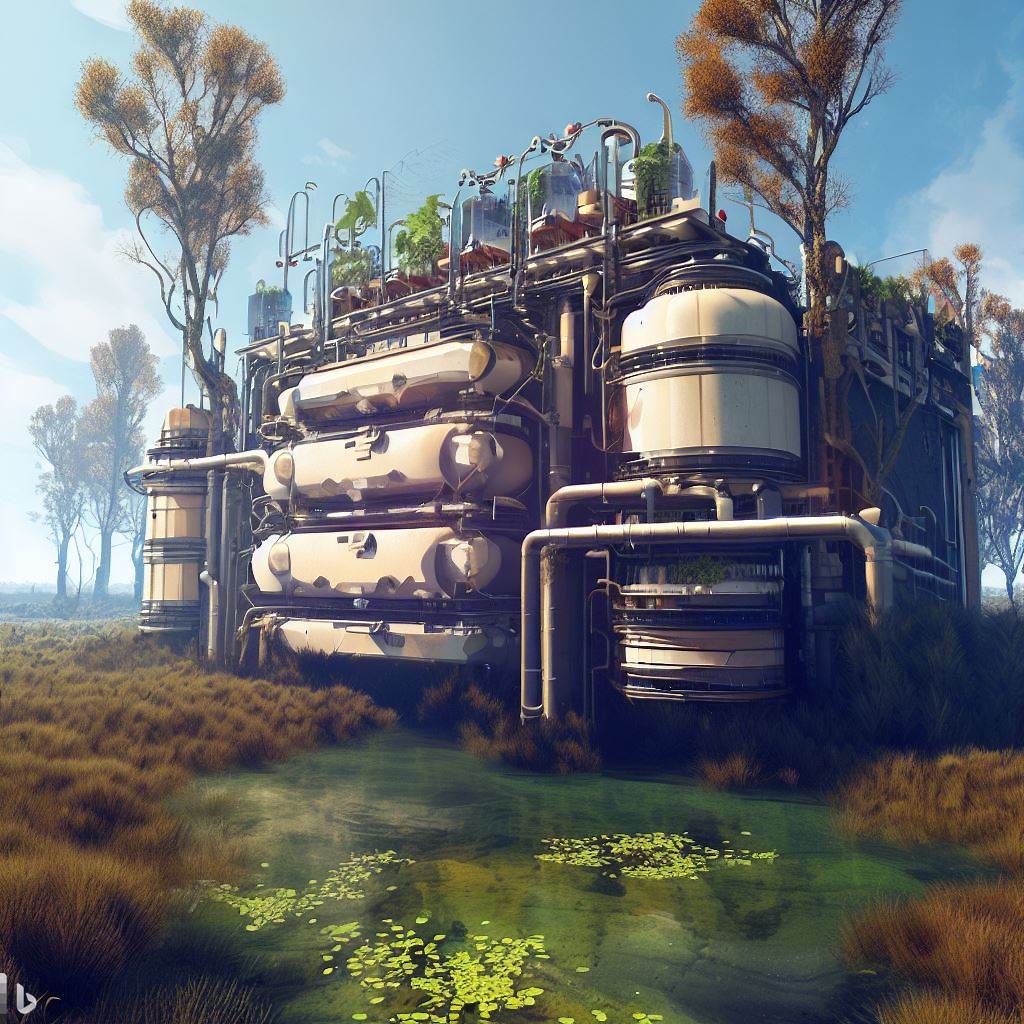